BICO News Feed
Discover the latest news from our business areas.
- August 27, 2024
- 12:10 pm
Streamlining Monoclonal Antibody Production: From Quality Control to Cost Management
Share on facebook
Share on twitter
Share on linkedin
Streamlining Monoclonal Antibody Production: From Quality Control to Cost Management
Monoclonal antibody production is a critical process in modern medicine, supporting the development of therapeutics that have proven invaluable across oncology, immunology, and virology (Mekala et al., 2024). Ensuring high-quality and cost-effective production involves rigorous quality control, optimizing production workflows, and adhering to regulatory standards. This guide covers best practices in monoclonal antibody production, explores their therapeutic applications, and provides strategies to streamline workflows and improve efficiency.
Quality Control and Best Practices for Monoclonal Antibody Production
Ensuring high-quality monoclonal antibody production requires rigorous quality control and adherence to best practices. For optimal results, scientists should aim to focus on the following key areas.
Mycoplasma Testing
Mycoplasma contamination can go undetected for long periods in cell cultures, compromising antibody quality (Fratz‐Berilla et al., 2020). Regular testing using methods like qPCR, indirect Hoechst staining, and culture isolation is essential to maintain production standards (Mycoplasma Testing, n.d.).
Monoclonal Verification
Verification of antibodies’ clonality is essential for adhering to regulatory requirements. Verification methods such as selective PCR and advanced tools like CYTENA’s UP.SIGHT (Fig. 1), which guarantees >99.99% clonal derivation, helps ensure the production of truly monoclonal antibodies (Babrak et al., 2017; Riba et al., 2020).
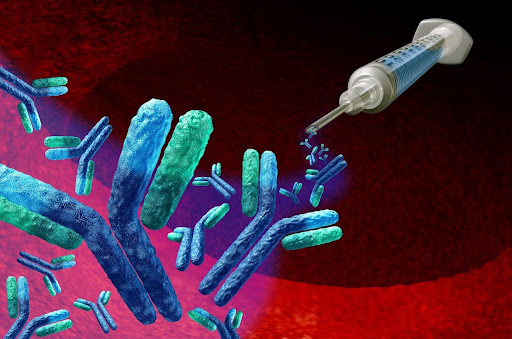
Figure 1. The UP.SIGHT has dual-imaging technology that supports clonality verification with documented proof to help scientists overcome regulatory hurdles.
Maximizing Antibody Yield
To obtain a high yield of monoclonal antibodies, it is essential to select the highest-producing clones, maintain stable hybridoma or cell line cultures, and optimize conditions like pH and temperature (Castillo et al., 1994; Wohlenberg et al., 2022). Tools like the UP.SIGHT and F.QUANT can be integrated to streamline clone selection and maximize production efficiency.
Cryopreservation
Effective cryopreservation is vital for maintaining cell viability. Cells should be properly suspended, rapidly frozen, and stored in liquid nitrogen in appropriate cryovials to ensure they can be recovered without losing functionality.
Regulatory Compliance
By following these guidelines and leveraging advanced technologies, labs can enhance the quality, yield, and compliance of monoclonal antibody production. For further insights into quality control and best practices for monoclonal antibody production, check out the full article.
Therapeutic Applications of Monoclonal Antibody Production
Monoclonal antibodies have revolutionized the treatment of various diseases, offering targeted and effective therapeutic options (Mekala et al., 2024). Here are the key therapeutic areas where monoclonal antibodies have made a significant impact:
Oncology
- Targeted Therapy: Monoclonal antibodies target specific proteins in cancer cells, blocking their growth and enhancing immune responses (Namboodiri & Pandey, 2011).
- Immune Checkpoint Inhibitors: Antibody therapies boost the immune system’s ability to fight cancer by inhibiting checkpoints (Shiravand et al., 2022).
- Antibody-Drug Conjugates (ADCs): ADCs deliver chemotherapy drugs directly to cancer cells, reducing damage to healthy tissues (Fu et al., 2022).
Immunology
- Autoimmune and Inflammatory Diseases: Monoclonal antibodies manage conditions such as rheumatoid arthritis and inflammatory bowel disease by preventing inflammatory pathways (Berger et al., 2002).
- Allergies: Monoclonal antibodies can reduce allergic reactions by modulating the immune response, increasing the threshold of tolerated allergens, and preventing severe reactions (Manti et al., 2021).
- Transplant Medicine: Monoclonal antibodies help prevent transplant rejection and manage post-transplant complications (Costanzo, 1996).
Virology
- Infectious Diseases: Monoclonal antibodies are frequently used to prevent or reduce the severity of infections like RSV, COVID-19, and Ebola by blocking viral entry and replication (Otsubo & Yasui, 2022).
Challenges in Therapeutic Applications
Despite their benefits, scientists frequently face challenges with antibody production workflows, including complex monoclonal antibody production processes, maintaining quality and consistency across batches, and potential immune reactions in patients (Sifniotis et al., 2019). Continuous research and advanced technologies are crucial to overcoming these hurdles and improving monoclonal antibody therapies.
To explore the therapeutic applications of monoclonal antibodies in more depth, check out our dedicated article.
Optimizing Monoclonal Antibody Production Workflows
Monoclonal antibody production is a multi-step process involving cell line development, culture optimization, and quality control to achieve a good yield of consistent, high-quality antibodies.
Key Steps in Production
- Cell Line Development: Select high-producing cell lines for antibody production.
- Upstream Cell Culture: Grow cells under optimized conditions to maximize yield.
- Harvesting and Purification: Techniques like filtration and chromatography are used to purify antibodies.
- Quality Control: Ensure rigorous testing throughout the process to maintain high-quality standards.
Optimization Strategies
- Automation: Reduces errors and enhances consistency in cell culture and purification processes (Fig. 2) (Holland & Davies, 2020).
- Single-cell Dispensing: Tools like CYTENA’s UP.SIGHT improves clone selection, ensuring high yield and stable antibody production.
- Picking Hits: High-throughput screening and advanced bioinformatics streamline the identification of top-performing clones (Bauer et al., 2023).
- Good Manufacturing Practice (GMP) Readiness: Implement GMP practices and GMP-compliant tools to ensure quality and compliance for scaling up to commercial production.
Quality Control
There are lots of potential pitfalls at every stage of cell line development. Therefore, keeping tabs on potential issues is essential for maximizing efficiency throughout the workflow. Robust quality control (QC) systems help ensure the reliability and consistency of cell lines, prevent contamination, verify genetic identity and stability, and ultimately maintain the productive capacity of cells.
Monoclonality
Verifying single-cell seeding is an early and essential QC step in cell line development. Demonstrable monoclonality is required for regulatory compliance and, therefore, for achieving success in therapy development. However, monoclonality can be challenging to prove, particularly with manual cell dispensing methods.
The UP.SIGHT from CYTENA images cells as they are being dispensed and after they have settled in the well, giving scientists robust evidence of monoclonality and allowing them to progress to the next stages of cell line development with confidence (Fig. 2). The UP.SIGHT ensures a >99.99% probability of clonal derivation and up to 80% clonal recovery rate, making it ideal for ambitious companies looking for a simple way to streamline their workflows.
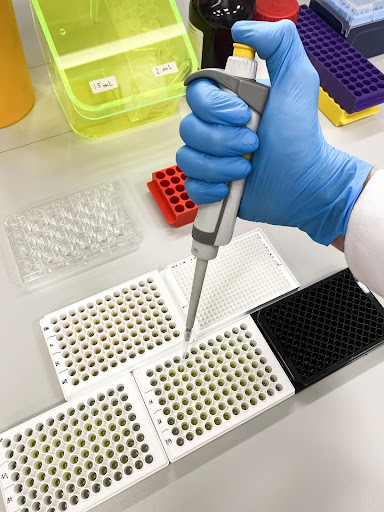
Figure 2. Assays can be streamlined, made more reproducible, and less prone to error by ditching the pipettes and opting for automated liquid handling systems instead.
By focusing on these strategies, labs can enhance efficiency and product quality in cost-effective monoclonal antibody production workflows. Read the full article to learn more about how advanced tools can support optimization in monoclonal antibody production workflows.
Conclusion
Optimizing monoclonal antibody production requires a strategic approach that integrates quality control, advanced technologies, and regulatory compliance. By focusing on these areas, laboratories can enhance antibody yield, maintain high quality, and reduce costs, ultimately supporting more effective and accessible therapies. Whether you are optimizing discovery workflows or scaling up production, implementing these best practices will help ensure the successful development of monoclonal antibodies for research, diagnostics, and therapeutic applications.
Visit our website to learn more about our comprehensive suite of instruments that offer end-to-end solutions for monoclonal antibody production. Ready to see the power of the UP.SIGHT for yourself? A comprehensive demo is just one click away.
References
- Babrak, L., McGarvey, J. A., Stanker, L. H., & Hnasko, R. (2017). Identification and verification of hybridoma-derived monoclonal antibody variable region sequences using recombinant DNA technology and mass spectrometry. Molecular Immunology, 90, 287–294. https://doi.org/10.1016/j.molimm.2017.08.014
- Bauer, J., Rajagopal, N., Gupta, P., Gupta, P., Nixon, A. E., & Kumar, S. (2023). How can we discover developable antibody-based biotherapeutics? Frontiers in Molecular Biosciences, 10, 1221626. https://doi.org/10.3389/fmolb.2023.1221626
- Berger, M., Shankar, V., & Vafai, A. (2002). Therapeutic Applications of Monoclonal Antibodies. The American Journal of the Medical Sciences, 324(1), 14–30. https://doi.org/10.1097/00000441-200207000-00004
- Castillo, F. J., Mullen, L. J., Grant, B. C., DeLeon, J., Thrift, J. C., Chang, L. W., Irving, J. M., & Burke, D. J. (1994). Hybridoma stability. Developments in Biological Standardization, 83, 55–64.
- Costanzo, M. R. (1996). New monoclonal antibodies. Current Opinion in Cardiology, 11(2). https://doi.org/10.1097/00001573-199603000-00014
- Fratz‐Berilla, E. J., Angart, P., Graham, R. J., Powers, D. N., Mohammad, A., Kohnhorst, C., Faison, T., Velugula‐Yellela, S. R., Trunfio, N., & Agarabi, C. (2020). Impacts on product quality attributes of monoclonal antibodies produced in CHO cell bioreactor cultures during intentional mycoplasma contamination events. Biotechnology and Bioengineering, 117(9), 2802–2815. https://doi.org/10.1002/bit.27436
- Fu, Z., Li, S., Han, S., Shi, C., & Zhang, Y. (2022). Antibody drug conjugate: The “biological missile” for targeted cancer therapy. Signal Transduction and Targeted Therapy, 7(1), 1–25. https://doi.org/10.1038/s41392-022-00947-7
- Holland, I., & Davies, J. A. (2020). Automation in the Life Science Research Laboratory. Frontiers in Bioengineering and Biotechnology, 8, 571777. https://doi.org/10.3389/fbioe.2020.571777
- Manti, S., Pecora, G., Patanè, F., Giallongo, A., Parisi, G. F., Papale, M., Licari, A., Marseglia, G. L., & Leonardi, S. (2021). Monoclonal Antibodies in Treating Food Allergy: A New Therapeutic Horizon. Nutrients, 13(7), 2314. https://doi.org/10.3390/nu13072314
- Mekala, J. R., Nalluri, H. P., Reddy, P. N., S.b., S., N.s., S. K., G.v.s.d., S. K., Dhiman, R., Chamarthy, S., Komaragiri, R. R., Manyam, R. R., & Dirisala, V. R. (2024). Emerging trends and therapeutic applications of monoclonal antibodies. Gene, 925, 148607. https://doi.org/10.1016/j.gene.2024.148607
- Mycoplasma testing. (n.d.). Culture Collections. Retrieved August 22, 2024, from https://www.culturecollections.org.uk/services/mycoplasma-testing/
- Namboodiri, A. M., & Pandey, J. P. (2011). Differential inhibition of trastuzumab- and cetuximab-induced cytotoxicity of cancer cells by immunoglobulin G1 expressing different GM allotypes. Clinical and Experimental Immunology, 166(3), 361–365. https://doi.org/10.1111/j.1365-2249.2011.04477.x
- Otsubo, R., & Yasui, T. (2022). Monoclonal antibody therapeutics for infectious diseases: Beyond normal human immunoglobulin. Pharmacology & Therapeutics, 240, 108233. https://doi.org/10.1016/j.pharmthera.2022.108233
- Riba, J., Schoendube, J., Zimmermann, S., Koltay, P., & Zengerle, R. (2020). Single-cell dispensing and ‘real-time’ cell classification using convolutional neural networks for higher efficiency in single-cell cloning. Scientific Reports, 10(1), 1193. https://doi.org/10.1038/s41598-020-57900-3
- Shiravand, Y., Khodadadi, F., Kashani, S. M. A., Hosseini-Fard, S. R., Hosseini, S., Sadeghirad, H., Ladwa, R., O’Byrne, K., & Kulasinghe, A. (2022). Immune Checkpoint Inhibitors in Cancer Therapy. Current Oncology, 29(5), 3044–3060. https://doi.org/10.3390/curroncol29050247
- Sifniotis, V., Cruz, E., Eroglu, B., & Kayser, V. (2019). Current Advancements in Addressing Key Challenges of Therapeutic Antibody Design, Manufacture, and Formulation. Antibodies, 8(2), 36. https://doi.org/10.3390/antib8020036
- Wohlenberg, O. J., Kortmann, C., Meyer, K. V., Schellenberg, J., Dahlmann, K., Bahnemann, J., Scheper, T., & Solle, D. (2022). Optimization of a mAb production process with regard to robustness and product quality using quality by design principles. Engineering in Life Sciences, 22(7), 484–494. https://doi.org/10.1002/elsc.202100172
More news
Stay updated
Get the latest first.
Subscribe and stay updated with the latest news!
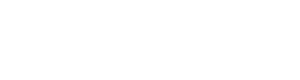

Copyright © 2024 BICO - The Bio Convergence Company - All rights reserved.
Contact us!
This site is protected by reCAPTCHA and the Google
Privacy Policy and
Terms of Service apply.