BICO News Feed
- November 11, 2024
- 2:56 pm
Modern Medicine Transformed: How Viral Vectors Drive Gene Therapy
Modern Medicine Transformed: How Viral Vectors Drive Gene Therapy
Gene therapy is transforming modern medicine, with viral vectors playing a central role in this advancement. These engineered viruses deliver therapeutic genetic sequences to specific cells, offering potential treatments for genetic disorders, cancers, and neurodegenerative diseases. This article will explore how viral vectors work, their applications, and the successes and challenges shaping the future of gene therapy in healthcare.
Mechanism of Delivery
Several methods exist for delivering gene therapies into cells. Each is better suited for particular applications, which must be considered early in development.
In-vivo and Ex-vivo
Gene therapy delivery can be broadly categorized based on how it is administered: directly into patients (in-vivo) or into cells that have been removed from a patient (autologous) or a donor (allogeneic) and then reintroduced after modification (ex-vivo) (Mendell et al., 2021). In-vivo methods can be more appropriate for transfecting postmitotic cells, which are more likely to express the therapeutic gene for extended periods, and for treating widespread disorders that don’t require tissue specificity. Ex-vivo approaches are often more suitable for targeting specific, easy-to-access cellular populations, such as immune cells, as in CAR T-cell therapies (Bui et al., 2024).
Adenovirus
Adenoviruses (Ads) have a high transfection efficiency and have been shown to yield prolonged production of their therapeutic instructions, with one study reporting the expression of CRISPR machinery for 200 days after adenovirus administration (Stephens et al., 2018).
Lentivirus
Lentiviruses have a single-stranded RNA genome and integrate into the host genome, making them more suitable for long-term expression that isn’t lost during cell division. This makes them more applicable for treating genetic disorders that require longstanding corrective treatment. These therapies carry the risk of insertional mutagenesis, but non-integrating lentiviral vectors are being investigated to combat this (Milone & O’Doherty, 2018).
Adeno-associated virus
Adeno-associated viruses (AAVs) are single-stranded DNA viruses that rarely integrate into the host genome and have broad tissue tropism. Luxturna, the first FDA-approved in-vivo gene therapy, was introduced in 2017 to treat inherited vision loss and uses AAV as its delivery method (Darrow, 2019). Despite their advantages, AAVs face challenges like genotoxicity from insertional mutagenesis and hepatotoxicity from the high doses needed for IV administration (Wang et al., 2024).
Applications
Gene therapies have a wide range of applications across various disease types, showing particular promise for neurodegenerative diseases, cancer, genetic disorders, and infectious diseases.
Cancer
CAR T-cell therapy is the most prominent example of gene therapy to treat cancer. This technology involves modifying a patient’s T cells to recognize and attack cancer cells (Feins et al., 2019).
Neurodegenerative Disorders
Many therapies in this area focus on introducing gene products that restore tissue function. For example, viral vectors are being investigated to introduce glutamic acid decarboxylase and glial cell line-derived neurotrophic factor, which help restore dopaminergic nerve function in Parkinson’s patients (Sudhakar & Richardson, 2019).
Infectious Disease
Viral vectors are increasingly used as the basis for vaccines, where the genetic information carried by the vector encodes an antigen that trains the immune system to attack a specific pathogen. Vaccines of this type are already on the market for COVID-19 and Ebola, and exciting progress is being made toward a vaccine for the Zika virus (Fig. 2) (Liu et al., 2024; McCann et al., 2022; Woodson & Morabito, 2024).
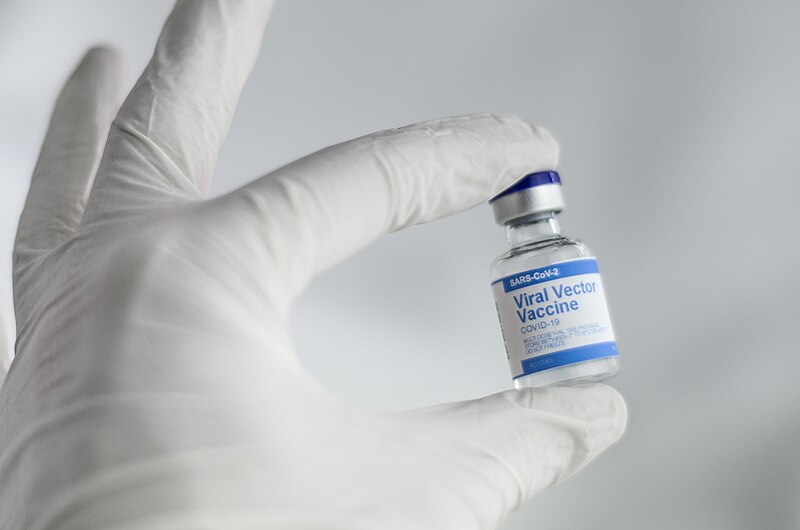
Genetic Disorders
Viral vectors can be used to carry corrective versions of genes to restore tissue function (Wang et al., 2024), and progress is being made to use viral vectors to deliver gene editing machinery to correct harmful mutations within the genome (Asmamaw Mengstie, 2022).
Success Stories
These success stories showcase the power of gene therapies to transform the lives of patients suffering from longstanding and acute conditions.
- Emily Whitehead was the first recipient of pediatric CAR T-cell therapy to treat acute lymphoblastic leukemia when she was seven years old. She was declared cancer-free a few weeks after receiving the treatment and remains so to this day (Bouzianas & Bouziana, 2024).
- Luxturna, the first FDA-approved in-vivo gene therapy using AAV, has restored and preserved vision in thousands of individuals who would otherwise have faced complete vision loss (Daley, 2021).
- In 2023, Roctavian became the first gene therapy to treat a bleeding disorder when it was approved by the FDA to treat severe hemophilia A. Roctavian is a one-time treatment, meaning patients no longer require regular injections to manage this disease (Samelson-Jones et al., 2024).
Challenges
Despite the promise of viral vectors for gene therapies, several bottlenecks prevent the widespread application of this methodology to more prevalent diseases.
Production
Different applications require customized development and production pipelines for viral vector production. Scaling up production comes with increased costs, and low yields remain a constant issue. Many processes struggle to obtain high ratios of full to empty capsids, which has direct implications for therapeutic dosage and patient safety (Wang et al., 2024).
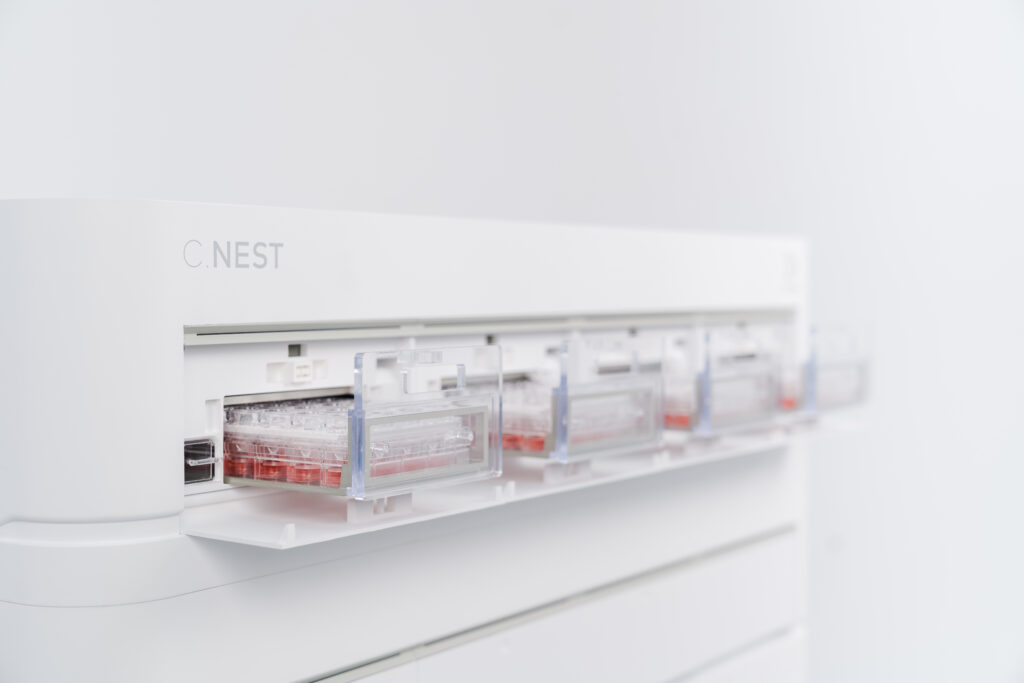
Regulatory Compliance
Potential side effects, limited long-term data, and the absence of standardized processes are prompting regulators to take a cautious approach to gene therapy approvals (Moffit et al., 2022; Research, 2024). One critical factor is demonstrating the monoclonality of cell lines that produce viral vectors; without proof of monoclonality, development timelines and regulatory approvals can face significant delays.
Conclusion and Future Directions
Gene therapy has shown tremendous potential, particularly through its successes in treating cancers and genetic disorders. Future efforts will focus on refining vector production and delivery methods to enhance safety, reduce off-target effects, and address immunogenicity challenges. Continued progress in vector development and regulatory frameworks will support wider accessibility, enabling gene therapy to transform healthcare across more diseases and patient populations.
Contact CYTENA today to learn more about our best-in-class instrumentation for viral vector production.
References
- Asmamaw Mengstie, M. (2022). Viral Vectors for the in Vivo Delivery of CRISPR Components: Advances and Challenges. Frontiers in Bioengineering and Biotechnology, 10, 895713.
- Bouzianas, D., & Bouziana, S. (2024). First pediatric B-acute lymphoblastic leukemia patient treated with anti-CD19 chimeric antigen receptor T-cell therapy: Long-term remission or early cure? Human Vaccines & Immunotherapeutics, 20(1), 2321678.
- Bui, T. A., Mei, H., Sang, R., Ortega, D. G., & Deng, W. (2024). Advancements and challenges in developing in vivo CAR T cell therapies for cancer treatment. eBioMedicine, 106, 105266.
- Daley, J. (2021). Four Success Stories in Gene Therapy. Nature, d41586-021-02737–7.
- Feins, S., Kong, W., Williams, E. F., Milone, M. C., & Fraietta, J. A. (2019). An introduction to chimeric antigen receptor (CAR) T‐cell immunotherapy for human cancer. American Journal of Hematology, 94(S1).
- Liu, X., Li, Z., Li, X., Wu, W., Jiang, H., Zheng, Y., Zhou, J., Ye, X., Lu, J., Wang, W., Yu, L., Li, Y., Qu, L., Wang, J., Li, F., Chen, L., Wu, L., & Feng, L. (2024). A single-dose circular RNA vaccine prevents Zika virus infection without enhancing dengue severity in mice. Nature Communications, 15(1), 8932.
- McCann, N., O’Connor, D., Lambe, T., & Pollard, A. J. (2022). Viral vector vaccines. Current Opinion in Immunology, 77, 102210.
- Mendell, J. R., Al-Zaidy, S. A., Rodino-Klapac, L. R., Goodspeed, K., Gray, S. J., Kay, C. N., Boye, S. L., Boye, S. E., George, L. A., Salabarria, S., Corti, M., Byrne, B. J., & Tremblay, J. P. (2021). Current Clinical Applications of In Vivo Gene Therapy with AAVs. Molecular Therapy, 29(2), 464–488.
- Milone, M. C., & O’Doherty, U. (2018). Clinical use of lentiviral vectors. Leukemia, 32(7), 1529–1541.
- Moffit, J. S., Blanset, D. L., Lynch, J. L., MacLachlan, T. K., Meyer, K. E., Ponce, R., & Whiteley, L. O. (2022). Regulatory Consideration for the Nonclinical Safety Assessment of Gene Therapies. Human Gene Therapy, 33(21–22), 1126–1141.
- Research, C. for B. E. and. (2024). Cellular & Gene Therapy Guidances. FDA.
- Samelson-Jones, B. J., Small, J. C., & George, L. A. (2024). Roctavian gene therapy for hemophilia A. Blood Advances, 8(19), 5179–5189.
- Stephens, C. J., Kashentseva, E., Everett, W., Kaliberova, L., & Curiel, D. T. (2018). Targeted in vivo knock-in of human alpha-1-antitrypsin cDNA using adenoviral delivery of CRISPR/Cas9. Gene Therapy, 25(2), 139–156.
- Sudhakar, V., & Richardson, R. M. (2019). Gene Therapy for Neurodegenerative Diseases. Neurotherapeutics: The Journal of the American Society for Experimental NeuroTherapeutics, 16(1), 166–175.
- Wang, J.-H., Gessler, D. J., Zhan, W., Gallagher, T. L., & Gao, G. (2024). Adeno-associated virus as a delivery vector for gene therapy of human diseases. Signal Transduction and Targeted Therapy, 9(1), 78.
- Woodson, S. E., & Morabito, K. M. (2024). Continuing development of vaccines and monoclonal antibodies against Zika virus. Npj Vaccines, 9(1), 91.
More news
Stay updated
Get the latest first.
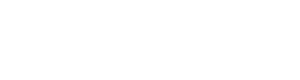
