BICO News Feed
- November 25, 2024
- 3:01 pm
The Ultimate Guide to Viral Vector Production: Applications, Quality Control, and Efficient Manufacturing Strategies
The Ultimate Guide to Viral Vector Production: Applications, Quality Control, and Efficient Manufacturing Strategies
Viral vectors are at the forefront of transformative treatments, enabling advancements in gene therapy and vaccines (Bulcha et al., 2021). These vectors deliver therapeutic genes and antigen-coding sequences, allowing for innovative approaches to treat genetic diseases and fight infectious pathogens. This blog explores the key applications, methodologies, and manufacturing strategies in viral vector production, highlighting the role of automation and cutting-edge tools in improving scalability and efficiency across production pipelines.
Key Applications
Viral vectors are driving the next generation of treatments, including gene therapies and vaccines.
Genetic diseases
Viral vectors can deliver corrective genes directly to diseased tissue and are being developed to carry gene-editing tools like CRISPR, which can reverse harmful disease-causing mutations (Asmamaw Mengstie, 2022). Gene therapies that use viral vectors have already been approved for various conditions, bringing life-changing relief to thousands of patients (Zhao et al., 2022).
Vaccines
Viral vectors are being used to deliver genetic information that encodes antigens, which train the body’s immune system to target pathogens. Therapies are already approved for viruses like COVID-19 and ebola and hold promise for many other conditions like HIV, Zika, and influenza (Travieso et al., 2022).
These advances have been made possible by critical technological advances, including automation.
Automation
Automation streamlines virtually all aspects of viral vector production, including the early stages of cell line development. It is far faster than manual cell culture methods and is less prone to error and contamination, which can put entire workflows and projects at risk. By adopting automation, researchers can save on resources and free up time to focus on other tasks, such as data analysis and troubleshooting.
The UP.SIGHT dual-imager and single-cell dispenser from CYTENA offers automated single-cell seeding to reduce risks in the early stages of viral vector development, ensuring projects start on a solid foundation (Fig. 1).
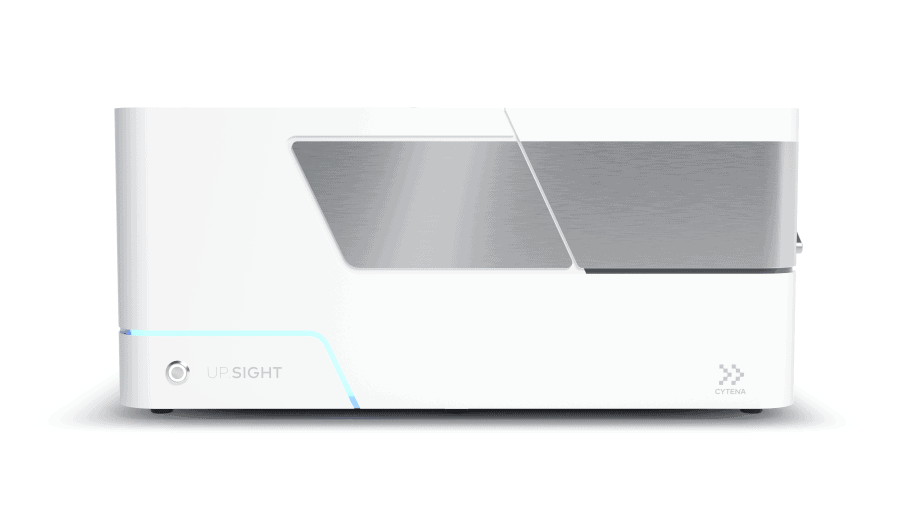
Read our full article on this topic for a deeper dive into the applications of viral vector production, including industry trends.
Gene Therapy Methods
In-vivo and Ex-vivo
In-vivo and ex-vivo are two broad categories of gene therapies. In-vivo describes gene therapies that are administered directly to the patient. These therapies are suitable for widespread disorders or to target cell types that are difficult to access or extract. Conversely, ex-vivo refers to removing cells from a donor or patient, modifying these cells to express a given construct, and administering them to the patient. This approach allows for easy-to-access cell populations like immune cells to be effectively targeted (Bulcha et al., 2021).
Virus Types
Adenoviruses (Ads), lentiviruses, and adeno-associated viruses (AAVs) are the primary viral vectors used to deliver therapeutic genetic material, and each comes with a set of advantages and disadvantages. Ads enable prolonged gene expression, with CRISPR machinery lasting up to 200 days in one instance (Stephens et al., 2018), but suffer from immunogenicity issues. Lentiviruses integrate into the host genome, allowing stable, long-term expression suited for treating genetic disorders, though they carry a risk of insertional mutagenesis (Milone & O’Doherty, 2018). AAVs, which rarely integrate into the genome, are used in Luxturna, the first FDA-approved in-vivo gene therapy, though challenges like hepatotoxicity remain (Darrow, 2019; Wang et al., 2024).
For a deeper understanding of gene therapy applications and success stories, consider reading our full article, which covers these and other topics in more detail.
Viral Vector Quality Control and Manufacturing Strategies
Challenges of Viral Vector Production
Viral vector is an umbrella term for diverse therapies with unique production needs. Ads, lentiviruses, and AAVs each require tailored production methods to ensure safety, efficacy, and scalability, with host-cell requirements adding further complexity (Bulcha et al., 2021; Wang et al., 2024). Scaling up the production of viral vectors is challenging due to low yields, especially for vectors like AAVs that require high dosages. Large-scale infrastructure investments may need modifications to remain compliant as regulatory standards evolve (Jiang & Dalby, 2023).
Strategies for Efficient Viral Vector Production
- Selecting Host Cells – Different cell lines are used to produce viral vectors. HEK293 cells, known for high transfection efficiency, are common in viral vector production, while the Baculovirus system uses insect cells for higher yields. Starting with producer cell lines can streamline vector development by reducing the need for plasmid transfections (Tridgett et al., 2024).
- Early Screening – Early screening for high-producing cell lines enhances resource efficiency by ensuring only suitable clones are taken forward for scaling. Screening multiple parameters, like empty-to-full capsid ratios, and confirming sufficient therapeutic gene expression are vital to ensuring product quality (Gimpel et al., 2021).
The S.NEST from CYTENA allows four plates in 24- and 96-formats to be cultured in parallel, meaning hundreds of clones can be cultured in suspension and screened on a small scale.
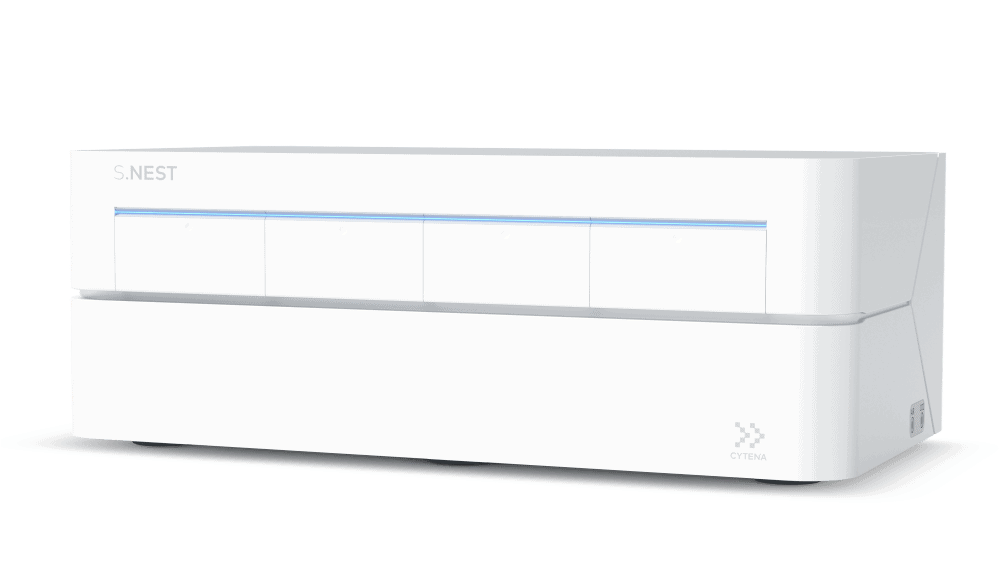
Quality Control
There are seemingly endless factors to consider during the production of viral vectors, including genomic titer, infectious titer, capsid titer, purity, and many more (Kontogiannis et al., 2024). One essential step to get right is ensuring the monoclonality of cells used for viral vector production. This can be accomplished using the UP.SIGHT from CYTENA.
To learn more about these topics, including emerging technologies that help researchers tackle the increasing complexity of viral vector production, read our full article.
Conclusion
Viral vector production is a cornerstone of modern gene therapy and vaccine development, driving treatments for genetic disorders and infectious diseases. By implementing advanced manufacturing techniques and rigorous quality control, researchers can enhance scalability, efficiency, and safety during production. Advancements in automation and AI, including optimizing coding sequences and predicting toxicity, will be crucial for addressing future therapeutic demands and ensuring these therapies fulfill their potential.
References
- Asmamaw Mengstie, M. (2022). Viral Vectors for the in Vivo Delivery of CRISPR Components: Advances and Challenges. Frontiers in Bioengineering and Biotechnology, 10, 895713.
- Bulcha, J. T., Wang, Y., Ma, H., Tai, P. W. L., & Gao, G. (2021). Viral vector platforms within the gene therapy landscape. Signal Transduction and Targeted Therapy, 6(1), 53.
- Darrow, J. J. (2019). Luxturna: FDA documents reveal the value of a costly gene therapy. Drug Discovery Today, 24(4), 949–954.
- Gimpel, A. L., Katsikis, G., Sha, S., Maloney, A. J., Hong, M. S., Nguyen, T. N. T., Wolfrum, J., Springs, S. L., Sinskey, A. J., Manalis, S. R., Barone, P. W., & Braatz, R. D. (2021). Analytical methods for process and product characterization of recombinant adeno-associated virus-based gene therapies. Molecular Therapy. Methods & Clinical Development, 20, 740–754.
- Jiang, Z., & Dalby, P. A. (2023). Challenges in scaling up AAV-based gene therapy manufacturing. Trends in Biotechnology, 41(10), 1268–1281.
- Kontogiannis, T., Braybrook, J., McElroy, C., Foy, C., Whale, A. S., Quaglia, M., & Smales, C. M. (2024). Characterization of AAV vectors: A review of analytical techniques and critical quality attributes. Molecular Therapy – Methods & Clinical Development, 32(3), 101309.
- Milone, M. C., & O’Doherty, U. (2018). Clinical use of lentiviral vectors. Leukemia, 32(7), 1529–1541.
- Stephens, C. J., Kashentseva, E., Everett, W., Kaliberova, L., & Curiel, D. T. (2018). Targeted in vivo knock-in of human alpha-1-antitrypsin cDNA using adenoviral delivery of CRISPR/Cas9. Gene Therapy, 25(2), 139–156.
- Travieso, T., Li, J., Mahesh, S., Mello, J. D. F. R. E., & Blasi, M. (2022). The use of viral vectors in vaccine development. Npj Vaccines, 7(1), 75.
- Tridgett, M., Mulet, M., Johny, S. P., Ababi, M., Raghunath, M., Fustinoni, C., Galabova, B., Fernández-Díaz, C., Mikalajūnaitė, I., Tomás, H. A., Kucej, M., Dunajová, L., Zgrundo, Z., Page, E., McCall, L., Parker-Manuel, R., Payne, T., Peckett, M., Kent, J., … Liu, Q. (2024). Lentiviral vector packaging and producer cell lines yield titers equivalent to the industry-standard four-plasmid process. Molecular Therapy – Methods & Clinical Development, 32(3), 101315.
- Wang, J.-H., Gessler, D. J., Zhan, W., Gallagher, T. L., & Gao, G. (2024). Adeno-associated virus as a delivery vector for gene therapy of human diseases. Signal Transduction and Targeted Therapy, 9(1), 78.
- Zhao, Z., Anselmo, A. C., & Mitragotri, S. (2022). Viral vector-based gene therapies in the clinic. Bioengineering & Translational Medicine, 7(1), e10258.
More news
Stay updated
Get the latest first.
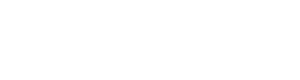
