Nyheter
- 2024-11-18
- 14:59
Strategies for Efficient Viral Vector Production for Gene Therapies
Strategies for Efficient Viral Vector Production for Gene Therapies
Viral vectors are essential tools in gene therapy, offering promising treatment options for various genetic diseases (Bulcha et al., 2021). However, scaling up production to meet growing demand introduces several challenges. Complexities in production, low yields, and stringent regulatory requirements make viral vector manufacturing a lengthy and resource-intensive process. This blog explores the critical barriers to efficient viral vector production and discusses practical strategies and emerging technologies that can help optimize and streamline the development process, paving the way for broader therapeutic applications.
Challenges of Viral Vector Production
The success of viral vectors in treating different genetic diseases has highlighted the potential for this method to treat more prevalent disorders. However, increasing the production of viral vectors to match the market’s demand comes with significant challenges.
Complexity
Viral vectors represent a diverse set of therapies, making them distinct from monoclonal antibodies for which there is standardized production guidance. Each delivery type, i.e., adenovirus (Ads), lentivirus, and adeno-associated virus (AAVs), has specific production requirements to ensure safety, efficacy, and scalability (Bulcha et al., 2021). Host-cell requirements add further complexity to the production process, with each coming with different pros and cons that affect immunogenicity and yield, among other factors (Wang et al., 2024).
Scaling up
The lower yields inherent to viral vector manufacturing necessitate even larger-scale protection to meet demands. AAV-based therapies often require high doses for a therapeutic effect, highlighting yield issues as a significant bottleneck for implementing viral vectors in the clinic (Fig. 1) (Jiang & Dalby, 2023). The unique demands of viral vector production workflows can lead to higher spending on large-scale infrastructure. This infrastructure may need adjustments or become obsolete as regulatory standards change, introducing an additional risk to scaling up production.
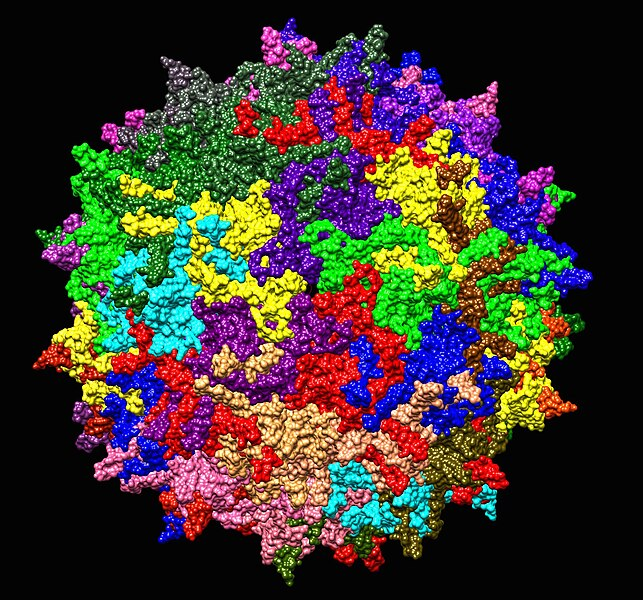
Strategies for Efficient Viral Vector Production
Strategies for more efficient viral vector production are continually emerging to meet market demands and facilitate large-scale manufacturing.
Selecting Appropriate Host Cells
HEK293 cells are widely used in viral vector production because they are a mammalian cell line with exceptionally high transfection efficiency. An alternative production method, the Baculovirus system, utilizes insect cell lines like Sf9, which can result in higher yields and simplified transfection protocols. Viral vector production can involve transfecting cells with multiple vectors encoding the viral capsid, packaging machinery, and the therapeutic genetic sequence. Beginning with stable cell lines, known as producer cell lines, already expressing some of these constructs can help to streamline development (Tridgett et al., 2024).
Early Screening
Screening for high-producing cell lines as early as possible in the production process can lead to more efficient resource allocation. Where possible, it’s important to screen for multiple parameters that give a better picture of product quality, such as the ratio between empty and full capsids. It is also important to ensure that the therapeutic genetic sequence has been successfully transfected and is expressed sufficiently (Gimpel et al., 2021).
Automation
Automation can be applied at virtually any stage of the viral vector production process. This includes single-cell seeding, cell culture, scaling up, and assays to determine clone performance and product quality. Automation allows processes to be run 24/7 and eliminates the need for manual input, which removes a significant source of contamination and lets researchers focus on other priorities (Holland & Davies, 2020).
Quality Control
Quality control (QC) is an essential part of the production process for any therapeutic. However, it may be particularly important when developing viral vectors, given the inherent safety issues associated with these approaches, the risk of contamination, and the stringent regulatory requirements applied to them (Kontogiannis et al., 2024). Ensuring the monoclonality of cell lines is an essential part of early QC in viral vector production.
The UP.SIGHT single-cell dispenser and dual-imager from CYTENA provides proof of monoclonality by imaging cells during dispensing and after they settle in the wells (Fig. 2). This streamlines and derisks development workflows while giving researchers peace of mind and allowing them to allocate their time to other tasks.
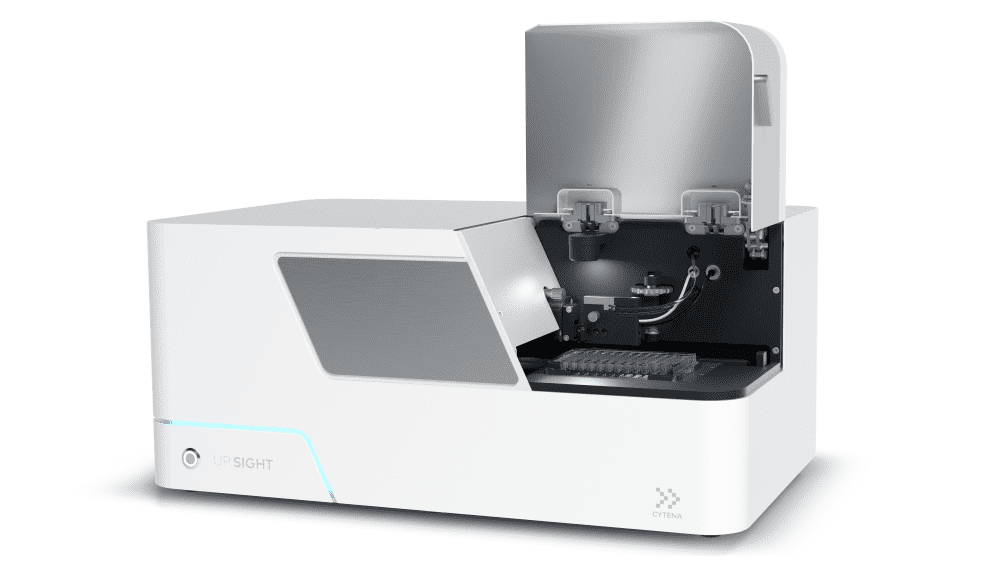
Emerging Technologies
Synthetic Biology
Researchers are investigating ways to reduce the need for multi-plasmid transfections, such as generating stable cell lines that produce viral vectors under the control of an inducible system (Lu et al., 2024). Producer cell lines eliminate the need for plasmid transfection, which becomes increasingly costly and challenging during large-scale production, especially when removing plasmid vectors from the production medium (Tridgett et al., 2024).
AI and Machine Learning
AI and machine learning are being used to optimize the coding sequences of different components of viral vectors (Wang et al., 2024). This may lead to greater stability and efficacy in some cases, which reduces the need for higher doses while maintaining the therapeutic effect. AI can also be used to predict the genotoxicity of viral vectors and to help analyze empty/full capsid ratios during production (Nishiumi et al., 2024; Schwarzer et al., 2021).
Conclusion
Viral vector manufacturing presents unique challenges due to its complexity, scaling demands, and stringent regulatory requirements. However, manufacturers can enhance efficiency and scalability by adopting innovative strategies like selecting suitable host cells, incorporating early screening, and leveraging automation. Emerging technologies like synthetic biology and AI are also proving instrumental in refining production and addressing safety concerns. As these advancements progress, they hold the potential to streamline viral vector manufacturing, ultimately supporting the broader application of gene therapies in healthcare.
Many researchers already use CYTENA’s high-end instrumentation to streamline their viral vector production workflows. Book a demo to test the UP.SIGHT for yourself or connect with our team today to optimize your production process with cutting-edge precision and efficiency.
References
- Bulcha, J. T., Wang, Y., Ma, H., Tai, P. W. L., & Gao, G. (2021). Viral vector platforms within the gene therapy landscape. Signal Transduction and Targeted Therapy, 6(1), 53.
- Gimpel, A. L., Katsikis, G., Sha, S., Maloney, A. J., Hong, M. S., Nguyen, T. N. T., Wolfrum, J., Springs, S. L., Sinskey, A. J., Manalis, S. R., Barone, P. W., & Braatz, R. D. (2021). Analytical methods for process and product characterization of recombinant adeno-associated virus-based gene therapies. Molecular Therapy. Methods & Clinical Development, 20, 740–754.
- Holland, I., & Davies, J. A. (2020). Automation in the Life Science Research Laboratory. Front Bioeng Biotechnol, 8(571777).
- Jiang, Z., & Dalby, P. A. (2023). Challenges in scaling up AAV-based gene therapy manufacturing. Trends in Biotechnology, 41(10), 1268–1281.
- Kontogiannis, T., Braybrook, J., McElroy, C., Foy, C., Whale, A. S., Quaglia, M., & Smales, C. M. (2024). Characterization of AAV vectors: A review of analytical techniques and critical quality attributes. Molecular Therapy – Methods & Clinical Development, 32(3), 101309.
- Lu, M., Lee, Z., Lin, Y., Irfanullah, I., Cai, W., & Hu, W. (2024). Enhancing the production of recombinant adeno‐associated virus in synthetic cell lines through systematic characterization. Biotechnology and Bioengineering, 121(1), 341–354.
- Moffit, J. S., Blanset, D. L., Lynch, J. L., MacLachlan, T. K., Meyer, K. E., Ponce, R., & Whiteley, L. O. (2022). Regulatory Consideration for the Nonclinical Safety Assessment of Gene Therapies. Human Gene Therapy, 33(21–22), 1126–1141.
- Nishiumi, H., Hirohata, K., Fukuhara, M., Matsushita, A., Tsunaka, Y., Rocafort, M. A. V., Maruno, T., Torisu, T., & Uchiyama, S. (2024). Combined 100 keV Cryo-Electron Microscopy and Image Analysis Methods to Characterize the Wider Adeno-Associated Viral Products. Journal of Pharmaceutical Sciences, S0022354924001230.
- Research, C. for B. E. and. (2024). Cellular & Gene Therapy Guidances. FDA.
- Schwarzer, A., Talbot, S. R., Selich, A., Morgan, M., Schott, J. W., Dittrich-Breiholz, O., Bastone, A. L., Weigel, B., Ha, T. C., Dziadek, V., Gijsbers, R., Thrasher, A. J., Staal, F. J. T., Gaspar, H. B., Modlich, U., Schambach, A., & Rothe, M. (2021). Predicting genotoxicity of viral vectors for stem cell gene therapy using gene expression-based machine learning. Molecular Therapy: The Journal of the American Society of Gene Therapy, 29(12), 3383–3397.
- Tridgett, M., Mulet, M., Johny, S. P., Ababi, M., Raghunath, M., Fustinoni, C., Galabova, B., Fernández-Díaz, C., Mikalajūnaitė, I., Tomás, H. A., Kucej, M., Dunajová, L., Zgrundo, Z., Page, E., McCall, L., Parker-Manuel, R., Payne, T., Peckett, M., Kent, J., … Liu, Q. (2024). Lentiviral vector packaging and producer cell lines yield titers equivalent to the industry-standard four-plasmid process. Molecular Therapy – Methods & Clinical Development, 32(3), 101315.
- Wang, J.-H., Gessler, D. J., Zhan, W., Gallagher, T. L., & Gao, G. (2024). Adeno-associated virus as a delivery vector for gene therapy of human diseases. Signal Transduction and Targeted Therapy, 9(1), 78.
More news
Håll dig uppdaterad
Få det senaste först.
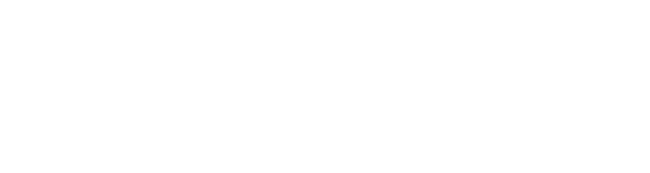
