Nyheter
- 2025-01-09
- 12:54
Advantages of C.WASH PLUS in Assay Automation: The ELISA Case
Enzyme-linked immunosorbent assays (ELISAs) are considered the gold standard for immunoassays and offer incredible specificity due to the inherent properties of antibody-antigen binding (Alhajj et al., 2024). Despite their reliability, traditional ELISA workflows can be time-consuming and error-prone when washing and pipetting steps are performed manually. Automation streamlines ELISA processes, enhancing accuracy and reproducibility. This ultimately improves the quality of results and their impact on diagnostics, research, and drug development. This article covers ELISA success stories, emerging technologies, the challenges (and solutions) associated with ELISA workflows, and the benefits of ELISA automation.
ELISA Success Stories
ELISAs have played a crucial role in some of the most significant breakthroughs in modern biomedical science. Let’s explore two of the remarkable advances humanity has made using this technology.
HIV Testing in the AIDS Epidemic
During the AIDS epidemic in the 1980s, clinicians lacked a reliable way to identify individuals carrying HIV. This represented a massive shortcoming in our ability to control the spread of the disease and implement effective public health interventions. The development of the first ELISA HIV test in 1985 marked a significant milestone in tracking and managing this health crisis (Alexander, 2016).
Prostate-Specific Antigen
Prostate-specific antigen (PSA) is one of the most enduringly useful cancer biomarkers and is fundamental for the non-invasive screening of prostate cancer. The discovery of PSA was translated into a diagnostic tool through ELISA technology, a development achieved in the mid-1980s through the collaboration of multiple laboratories (Catalona, 2014; Stamey et al., 1987).
Primary ELISA Applications
ELISA’s high sensitivity for virtually any antigen makes it a valuable and versatile tool for various applications across clinical and research settings. It is essential for disease diagnosis, including detecting infectious diseases like SARS-CoV-2 and autoimmune disorders (Deutschmann et al., 2020; MacMullan et al., 2020).
ELISAs are routinely used in research settings for protein detection and quantification. Specific applications include cytokine and chemokine assays, which are important for immunology and oncology research (Stefura et al., 2019; Wang et al., 2024). In drug development and manufacturing, ELISAs are essential for screening, quantifying therapeutic proteins, ensuring product quality, and monitoring immune responses during preclinical and clinical trials (Manak et al., 2024; Wadhwa et al., 2015). In addition to research and clinical settings, ELISA plays important roles in forensics, food safety, and environmental monitoring (Alhajj et al., 2024; Jaria et al., 2020).
ELISA Technological Developments
ELISA technology was invented in 1971 and has seen significant technological advancements in the decades since (Aydin, 2015). Notable innovations include multiplexed ELISAs, the use of nanoparticles to enhance sensitivity, and digital ELISA, which provides ultrasensitive single-molecule analyte detection (Li et al., 2022; Tabatabaei et al., 2021; Yi et al., 2022).
These recent advancements in ELISA assays demonstrate their continued relevance even five decades later, reinforcing their lasting importance in several fields.
Challenges in ELISA Workflows
While essential to modern clinical and research applications, ELISA workflows face persistent challenges. Firstly, ELISAs are incredibly labor-intensive to perform by hand and manual pipetting errors and inadequate washing can lead to contamination and experimental failure, increasing costs and delaying research. Furthermore, long incubation times can reduce throughput in high-demand settings.
As assays become more sensitive and specific, they don’t necessarily become more robust. Even advanced plate-based ELISAs remain complex, error-prone procedures that are highly dependent on the operator’s skills, particularly in pipetting and plate washing. Operator variability can increase result variability, reduce reproducibility, and introduce false positives or negatives, sometimes without detection. Advances in workflow automation place greater demands on precise liquid handling for ELISA assays.
Workflow inconsistencies waste time, money, and resources. Repeating experiments to verify ambiguous results not only delays progress but also drives up costs, especially given the high price of primary antibodies.
Quality of Biological Samples
Poor sample quality can significantly impact assay performance.
Solutions:
- Perform relevant sample quality control (QC) to ensure integrity.
- Avoid repeated freeze-thaw cycles, which can alter antigen concentrations.
Reagent Variability and Stability
Not all reagents perform equally, and their effectiveness can degrade over time.
Solutions:
- Different lots of primary or secondary antibodies may have different binding affinities. Use the same batch of all critical components across individual projects.
- Enzyme-linked antibodies and substrates are sensitive to temperature, light, and freeze-thaw cycles. Minimize reagent degradation by aliquoting reagents in single-use aliquots.
Inconsistent Reagent Dispensing
Variations in pipetting technique can introduce significant inconsistencies.
Solutions:
- Improper pipette tip changes or reagent handling can introduce cross-contamination artifacts. Use non-contact pipetting or tip-free dispensing.
- Distractions, daydreaming, thumb cramps and shaky hands can all lead to inaccuracies in liquid dispensing. We’ve all been there; consider automating the dispensing process.
Lag Time Between Workflow Steps
The longer the time between treatment of the first well and the last well of a plate, the more likely temperature differences and evaporation will impact the assay and its results
Solutions:
- Minimize time gaps between the first and last wells treated.
- Consider automated dispensing solutions which provide the speed and accuracy to avoid these issues.
nconsistent Washing and Reagent Removal
Insufficient washing can lead to high background noise or carryover effects.
Solutions:
- Aim for robust and consistent washing across all wells.
- Avoid harsh dispensing which can physically strip away immobilized analytes or antibodies
The Cost of Error in ELISA Workflows
Keeping these sources of variation in check is particularly important as ELISA workflows also suffer from high reagent costs, meaning that high variability and a lack of scalability can strain laboratory budgets and extend project timelines.
While the probability of an ELISA failure can never be brought to zero, relying on manual pipetting guarantees an inherently slow and error-prone workflow. Fortunately, the inter- and intra-experimental variability can be drastically decreased using lab automation. Automated laboratory instruments excel at one thing: delivering consistent results any time, all the time, from 8 am to 6 pm, independently of their users’ years of lab experience, pipetting skills, caffeine intake, or Monday morning or Friday afternoon mood.
ELISA Automation
Broad adoption of lab automation is a transformative development across virtually all life science applications. It is particularly useful for performing highly sensitive and repetitive tasks like ELISAs, which require multiple washing and incubation steps and can take a full day to pipette manually. Pipetting mistakes during ELISA workflows can lead to costly delays and wasted reagents.
Automating the different steps of an ELISA assay ensures that experiments are performed accurately and consistently. The improved efficiency enables smaller-scale experiments, reducing the number of wash cycles to save time, lower reagent costs, and minimize liquid waste. Automation also frees researchers from performing lengthy and tedious protocols that carry the risk of repetitive strain injuries (Wu et al., 2014).
The C.WASH PLUS from CYTENA streamlines your ELISA workflow by automating both the dispensing and removal of wash buffers.
- High Wash Efficiency: As a centrifugal washer, the C.WASH PLUS has an impressive wash efficiency of >99.99% (Fig. 1).
- Eco-Friendly, Tip-Free Dispensing: As a non-contact dispenser, the C.WASH PLUS saves your lab thousands of plastic tips a year (Fig. 2).
- Versatile Two-in-One Solution: Compatible with 96-, 384-, and 1536-well plates, the C.WASH PLUS seamlessly automates ELISA plate washing across a range of formats.
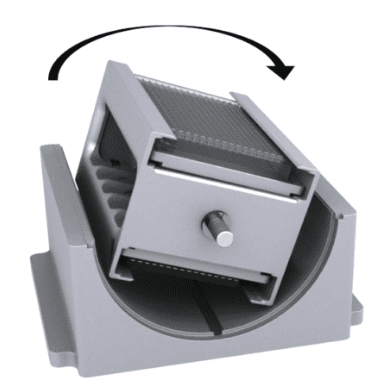
Conclusion
ELISA has been a fundamental technique for over fifty years, and recent technological advancements, such as microfluidics and CRISPR-ELISA, demonstrate its continued relevance and importance (Kweon et al., 2024; Li et al., 2022). These innovations ensure that ELISA will remain a central tool in scientific research for decades to come.
Despite their utility and sensitivity, ELISA assays can suffer from their multistep, error-prone procedures, leading to potentially damaging variability and artifacts. Automating ELISA dispensing and washing steps goes a long way toward increasing the consistency of your results but also the throughput of your lab by accelerating repetitive steps and freeing up your time.
Innovations like the C.WASH PLUS from CYTENA give a new spin to ELISA washing steps, combining precision dispensing with efficient removal of liquids, while minimizing cross-contamination and plastic waste. Consistent and reproducible ELISA workflows help you and your peers feel confident when interpreting biological data and make progress towards your research goals.
Contact CYTENA today to discover how the C.WASH PLUS can simplify your ELISA assays, along with other molecular or cell-based assays, while making your lab work more efficient and hassle-free.
References
Alexander, T. S. (2016). Human Immunodeficiency Virus Diagnostic Testing: 30 Years of Evolution. Clinical and Vaccine Immunology: CVI, 23(4), 249–253.
Alhajj, M., Zubair, M., & Farhana, A. (2024). Enzyme Linked Immunosorbent Assay. In StatPearls. StatPearls Publishing.
Aydin, S. (2015). A short history, principles, and types of ELISA, and our laboratory experience with peptide/protein analyses using ELISA. Peptides, 72, 4–15.
Catalona, W. J. (2014). History of the discovery and clinical translation of prostate-specific antigen. Asian Journal of Urology, 1(1), 12–14.
Deutschmann, C., Roggenbuck, D., Schierack, P., & Rödiger, S. (2020). Autoantibody testing by enzyme-linked immunosorbent assay-a case in which the solid phase decides on success and failure. Heliyon, 6(1), e03270.
Jaria, G., Calisto, V., Otero, M., & Esteves, V. I. (2020). Monitoring pharmaceuticals in the aquatic environment using enzyme-linked immunosorbent assay (ELISA)—A practical overview. Analytical and Bioanalytical Chemistry, 412(17), 3983–4008.
Kweon, O. J., Yoon, S., Choe, K. W., Kim, H., Lim, Y. K., & Lee, M.-K. (2024). Performance evaluation of microfluidic microplate-based fluorescent ELISA for qualitative detection of SARS-CoV-2–specific IgG and IgM. Scientific Reports, 14(1), 18200.
Li, N., Chinthalapally, M., Holden, V. K., Deepak, J., Dhilipkannah, P., Fan, J. M., Todd, N. W., & Jiang, F. (2022). Profiling Plasma Cytokines by A CRISPR-ELISA Assay for Early Detection of Lung Cancer. Journal of Clinical Medicine, 11(23), 6923.
MacMullan, M. A., Ibrayeva, A., Trettner, K., Deming, L., Das, S., Tran, F., Moreno, J. R., Casian, J. G., Chellamuthu, P., Kraft, J., Kozak, K., Turner, F. E., Slepnev, V. I., & Le Page, L. M. (2020). ELISA detection of SARS-CoV-2 antibodies in saliva. Scientific Reports, 10(1), 20818.
Manak, M., Gagnon, L., Phay-Tran, S., Levesque-Damphousse, P., Fabie, A., Daugan, M., Khan, S. T., Proud, P., Hussey, B., Knott, D., Charlton, S., Hallis, B., Medigeshi, G. R., Garg, N., Anantharaj, A., Raqib, R., Sarker, P., Alam, M. M., Rahman, M., … Carless, J. (2024). Standardised quantitative assays for anti-SARS-CoV-2 immune response used in vaccine clinical trials by the CEPI Centralized Laboratory Network: A qualification analysis. The Lancet Microbe, 5(3), e216–e225.
Stamey, T. A., Yang, N., Hay, A. R., McNeal, J. E., Freiha, F. S., & Redwine, E. (1987). Prostate-specific antigen as a serum marker for adenocarcinoma of the prostate. The New England Journal of Medicine, 317(15), 909–916.
Stefura, W. P., Graham, C., Lotoski, L., & HayGlass, K. T. (2019). Improved Methods for Quantifying Human Chemokine and Cytokine Biomarker Responses: Ultrasensitive ELISA and Meso Scale Electrochemiluminescence Assays. In P. Lympany & M. G. Jones (Eds.), Allergy (Vol. 2020, pp. 91–114). Springer New York.
Tabatabaei, M. S., Islam, R., & Ahmed, M. (2021). Applications of gold nanoparticles in ELISA, PCR, and immuno-PCR assays: A review. Analytica Chimica Acta, 1143, 250–266.
Wadhwa, M., Knezevic, I., Kang, H.-N., & Thorpe, R. (2015). Immunogenicity assessment of biotherapeutic products: An overview of assays and their utility. Biologicals, 43(5), 298–306.
Wang, H. R., Zhang, Y., Mo, Y. J., Zhang, Z., Chen, R., Lu, X. B., & Huang, W. (2024). Reshaping tumor microenvironment by regulating local cytokines expression with a portable smart blue-light controlled device. Communications Biology, 7(1), 916.
Yi, J., Gao, Z., Guo, Q., Wu, Y., Sun, T., Wang, Y., Zhou, H., Gu, H., Zhao, J., & Xu, H. (2022). Multiplexed digital ELISA in picoliter droplets based on enzyme signal amplification block and precisely decoding strategy: A universal and practical biodetection platform. Sensors and Actuators B: Chemical, 369, 132214.
More news
Håll dig uppdaterad
Få det senaste först.
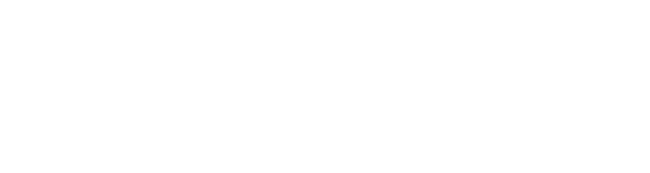
